Nicholas Strausfeld, Ph.D.
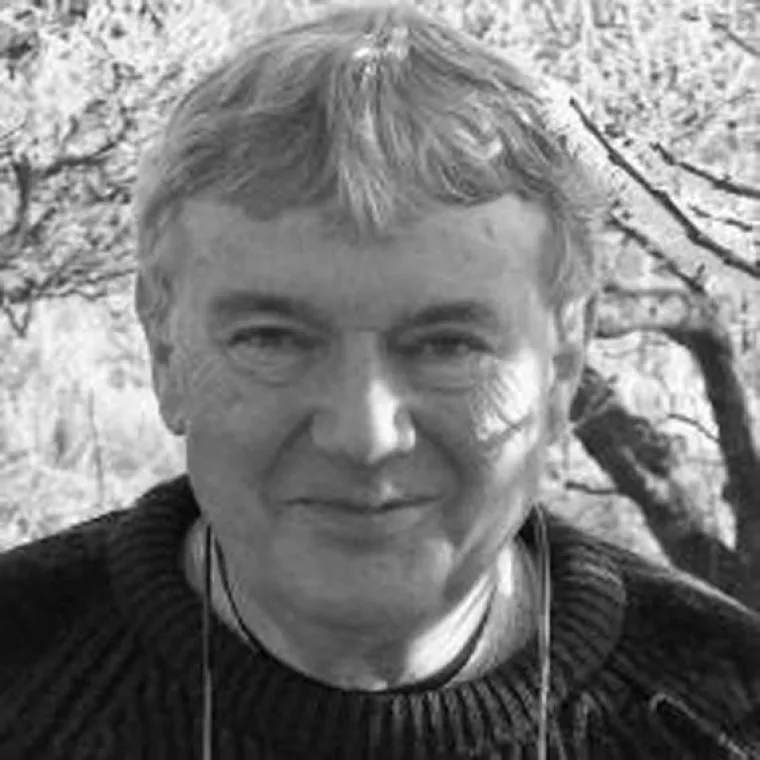
Rm 415 Gould-Simpson
Research Interests:
Areas of research pursued in my laboratory focus on the functional organization of the arthropod visual system and the evolution of brains; the latter focusing on the identification of evolutionarily conserved ground patterns of neuronal organization of centers mediating visual perception, allocentric memory, and action selection.
Arthropoda is the most species-rich phylum offering enormous challenges to systematists with respect to resolving taxonomic relationships within this group and reconstructing its evolutionary history. An attempt to resolve the phylogenetic relationships amongst the major arthropod groups has traditionally used morphological characters. In recent years, the ascendance of molecular phylogenomics, relying on molecular sequence data to infer evolutionary relationships, has all but displaced morphology-based studies except in the case of determining phylogenies amongst fossil taxa. However, as shown by the Swedish anatomist Nils Holmgren in 1910, comparisons of brain organization can also suggest evolutionary relationships, particularly amongst the invertebrates. Studies from my laboratory, begun in 1995, confirmed that certain brain morphologies define specific groups of arthropods irrespective of differences in their external morphologies.
It is now known that neural architectures that typify many of brain centers are highly conserved. They can be used as data points for Hennigian cladistics, which provides a relatively unbiased route to resolving such phylogenetic relationships. Many well-defined structural characters are defined by various histological and immunocytological methods. These include types of axonal projections, cell body arrangements, and architectures typifying synaptic neuropils. Each of these characters can be scored as present or absent in more than 70 taxa representing almost all arthropod groups, along with outgroups belonging to the Phylum Lophotrochozoa, such as planarians, annelids and nemerteans. Computational tools, such as PAUP or Mesquite, can be used to reconstruct taxonomic relatedness by determining which taxa share the most and which the least these characters and all the intermediates between. The resultant trees (cladograms) can be interrogated to determine what characters, or clusters of characters are present in what taxa, whether their presence has evolved by convergence or whether their shared presence is due to genealogical correspondence (homology).
For example, the presence of three successive visual centers in chelicerates and mandibulates is clearly convergent whereas the presence of mushroom bodies, paired centers in the forebrain of arthropods, is likely to be an ancient feature that evolved before the divergence of arthropods and lophotrochozoans. An unexpected finding from neural cladistics resolves Hexapoda originating from a malacostracan-like ancestor, agree with the results of molecular phylogenetics from other laboratories.
Neural cladistics challenges long-held assumptions, one being that simple fresh water crustaceans known as branchiopods gave rise to the hexapods. Branchiopods have very simple bipartite brains and possess only two optic neuropils in contrast to the three or more nested optic neuropils of insects and malacostracans. A branchiopod-like ancestry of hexapods implies that similarities of brain organization in insects and malacostracans would have evolved by convergence. However, discoveries from lower Cambrian rocks in 2011 of brains in fossil stem arthropods that existed before the appearance of branchiopods demonstrate animals possessed compound eyes supplying three nested optic neuropils and a tripartite brain. This alone suggests that many of the circuits observed in the optic centers of recently evolved taxa, such as Drosophila, are likely to have been inherited largely unchanged from an ancestor 520 million years ago. New observations of stem arthropod called megachierans, animals equipped long pincer-like head appendages and two pairs of compound eyes, demonstrated they had an arachnid-type brain. Thus, two of the ground patterns found today in extant mandibulates and chelicerates have not changed for over half a billion years.
Theodosius Dobzhansky (1900-1975), one of the founders of the modern evolutionary synthesis, insisted that "Nothing in biology makes sense except in the light of evolution." This dictum should resonate in the laboratories of brain researchers everywhere, but it rarely does. However, many working on the brains of arthropods follow the trajectory set by Nils Holmgren and see these brains in an evolutionary context. And there is some indication that the wider community of neuroscientists is taking some notice that iconic centers in insects, such as the mushroom bodies, or midline neuropils called central complexes, may be derived from ancient neuronal ground patterns that are shared by homologous centers in vertebrates. The candidate centers are, respectively, the hippocampus and basal ganglia . The view that arthropods have simple brains is slowly being displaced by the realization that they are anything but simple: that the elaborate organization of the arthropod brain suggests circuits and functions that typify the brains of vertebrates.
Career
I graduated from University College London, receiving my Ph.D. in 1968. After a year‘s postdoctoral work at the University of Frankfurt as an Alexander von Humboldt Fellow I was recruited in 1970 by Max Planck Institute for Biological Cybernetics in Tübingen, and from there moved to the European Molecular Biology Laboratory as a group leader in 1975. After habilitating at the University of Frankfurt in 1986, the Division of Neurobiology at the University of Arizona took me in as a “full” professor. My career in the USA has been quite eventful. I received a John Simon Guggenheim Memorial Foundation Fellowship in 1994, a John D. and Catherine T. MacArthur Foundation Fellowship in 1995, an Alexander von Humboldt Senior Research Prize in 2001, and a Volkswagen Stiftung Visiting Professorship in 2009. I was elected a Fellow of the Royal Society of London in 2002. Presently I’m a Regents Professor in the Department of Neuroscience and Director of the University of Arizona's Center for Insect Science. I have sole-authored two books: “Atlas of an Insect Brain” (Springer Verlag, 1976) and “Arthropod Brains: Evolution, Functional Elegance, and Historical Significance” (Harvard University, Belknap Press, 2012).
Selected Publications:
Strausfeld NJ. 2009. Brain organization and the origin of insects: an assessment. Proc Biol Sci 276:1929-1937.
Strausfeld NJ, Sinakevitch I, Brown SM, Farris SM. 2009. Ground plan of the insect mushroom body: functional and evolutionary implication. J Comp Neurol 513:265-291.
Strausfeld NJ 2010. Neurons and circuits that contribute to the detection of directional motion across the retina of the fly. Handbook of Brain Microcircuits. Eds: Gordon M. Shepherd and Sten Grillner. Oxford University Press.
Strausfeld NJ, Andrew DR. 2011. A new view of insect-crustacean relationships I. Inferences from neural cladistics and comparative neuroanatomy. Arthropod Struct Dev. 40:276-288.
Strausfeld NJ. 2011. Some observations on the sensory organization of the stem crustacean Waptia fieldensis Walcott. Palaeontographica Canadiana 31:157-268.
Ma X, Hou X, Edgecombe GD, Strausfeld NJ. 2012. Complex brain and optic lobes in an early Cambrian arthropod. Nature. 490:258-261.
Mu L, Ito K, Bacon JP, Strausfeld NJ. 2012. Optic glomeruli and their inputs in Drosophila share an organizational ground pattern with the antennal lobes. J Neurosci. 32:6061-6071.
Lin C, Strausfeld NJ. 2012. Visual inputs to the mushroom body calyces of the whirligig beetle Dineutus sublineatus: modality switching in an insect. J Comp Neurol. 520:2562 2574.
Phillips-Portillo J, Strausfeld NJ. 2012. Representation of the brain's superior protocerebrum of the flesh fly, Neobellieria bullata, in the central body. J Comp Neurol. 520:3070-3087.
Andrew DR, Brown SM, Strausfeld NJ. 2012.The minute brain of the copepod Tigriopus californicus supports a complex ancestral ground pattern of the tetraconate cerebral nervous systems. J Comp Neurol. 520:3446-3470.
Wolff G, Harzsch S, Hansson BS, Brown S, Strausfeld NJ. 2012. Neuronal organization of the hemiellipsoid body of the land hermit crab, Coenobita clypeatus: correspondence with the mushroom body ground pattern. J Comp Neurol. 520:2824-2846.
Lin C, Strausfeld NJ. 2013. A precocious adult visual center in the larva defines the unique optic lobe of the split-eyed whirligig beetle Dineutus sublineatus. Frontiers in Zoology 10:1-10.
Strausfeld NJ, Hirth F. 2013. Deep homology of arthropod central complex and vertebrate basal ganglia. Science. 340:157-161.
Tanaka G, Hou X, Ma X, Edgecombe GD, Strausfeld NJ. 2013. Chelicerate neural ground pattern in a Cambrian great appendage arthropod. Nature. 2013 Oct 17;502(7471):364-367.
Strausfeld NJ, Hirth F. 2013. Homology versus convergence in resolving transphyletic correspondences of brain organization. Brain Behav Evol. 82:215-219.
Mu L, Bacon, JP, Ito K, Strausfeld NJ. 2014. Responses of Drosophila giant descending neurons to visual and mechanical stimuli. J. E. B. 217, 2121-2129.
Ito K, Shinomiya K, Ito M, Armstrong D, Boyan G, Hartenstein V, Harzsch S, Heisenberg M, Homberg U, Jenett A, Keshishian H, Restifo L, Rössler W, Simpson J, Strausfeld NJ, Strauss R, Vosshall LB. 2014. A systematic nomenclature for the insect brain. Neuron 81, 755-765 .
Ma X, Cong P, Hou X, Edgecombe GD, Strausfeld NJ. 2014. An exceptionally preserved arthropod cardiovascular system from the early Cambrian. Nat Comm 5, 3560.